What was it like when the first atoms formed?
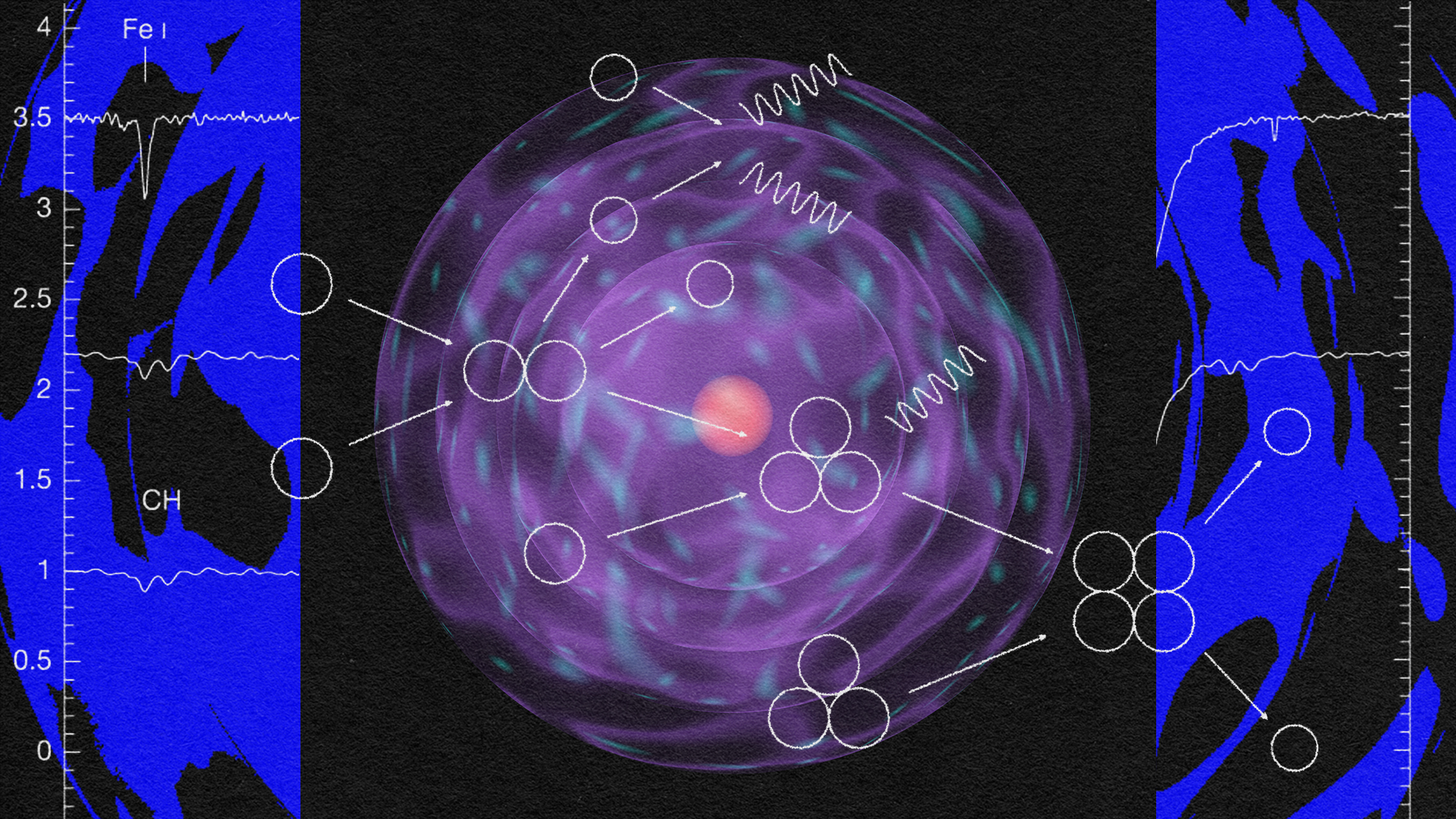
- Although it only took minutes for the first stable atomic nuclei to form in the Universe after the hot Big Bang, it takes hundreds of thousands of years to pass before neutral atoms come to stably exist.
- It’s primarily because the Universe was so hot in the early stages of the Big Bang, and photons are so much more numerous than atoms. It isn’t until the Universe cools off that atoms can stably form.
- And yet, even with that, it isn’t just as simple as “electrons join up with atomic nuclei, and atoms form.” There’s some intricate atomic physics that makes it happen, otherwise it would’ve taken even longer!
When it comes to our world, our Solar System, and all the planets, stars, and galaxies we can detect within our Universe, we find that they’re all made up of the same ingredients: atoms. And from the subatomic particles that bind together to make those atoms — electrons and atomic nuclei — we find that neutral atoms in turn interact and link up to form more complex structures: simple and complex molecules, some of which can eventually give rise to macroscopic structures and even life. It’s one of the most impressive facts about the Universe: that the laws and contents of the Universe exist in such a way that they admit even the possibility of complex structure. When we look, that’s precisely what we find within it today.
But for hundreds of thousands of years, dating from the instant of the hot Big Bang, it was impossible to form even a single atom. Even though it only takes minutes to form atomic nuclei and leave the Universe in a state where electrons and those nuclei are steeped in a mere background bath of photons and neutrinos (and antineutrinos), it takes hundreds of thousands of years for atoms to stably form. It takes a huge amount of cosmic evolution, and a number of important evolutionary steps, in order to create them. Here’s the story of how the first atoms were formed.

By the time the Universe is four minutes old, it’s already done fusing all the atomic nuclei it can fuse in this hot, dense, early state. There are no more free neutrons; they’ve all been incorporated into heavier nuclei. These include:
- Helium-4 (two protons and two neutrons),
- Deuterium (one proton and neutron),
- Helium-3 (two protons and one neutron) and Tritium (one proton and two neutrons),
- and Lithium-7 (three protons and four neutrons) and Beryllium-7 (four protons and three neutrons).
That’s pretty much it.
There are exactly enough free electrons to keep the Universe electrically neutral, balancing out the number of protons precisely. Even as the radioactive elements (tritium and beryllium-7) decay, the total amount of electric charge is still conserved, with the number of electrons present always balancing the number of protons present.
Despite the fact that we have a hot, dense state, and lots of atomic nuclei bouncing around, there are no further nuclear reactions that proceed from this point.
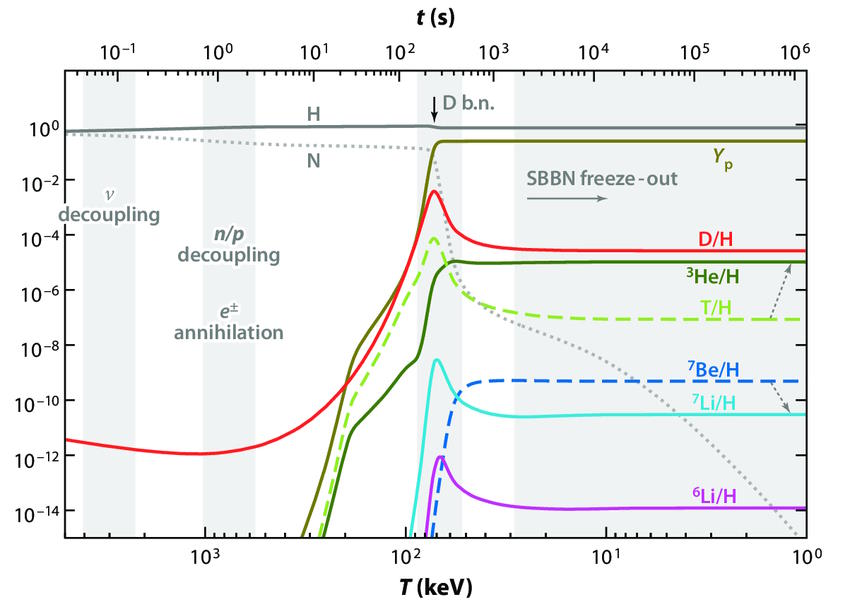
The reason for this is simple: there isn’t enough energy for these nuclei to fuse together into heavier combinations. However, at the same time, there’s too much energy for electrons to bind to them and form atoms. In fact, there’s way too much energy to form neutral atoms. Within this primeval bath of charged subatomic particles, the photons, the particles that are the quanta of light, scatter off of both the electrons and the atomic nuclei continuously. Electrons and nuclei can attempt to form neutral atoms, but it’s far too hot or energetic for them to form without immediately being blasted apart.
The reason why has everything to do with both the number of photons present — more than a billion for each atomic nucleus that exists — and also the extraordinarily hot temperatures present at early times. By the time the Universe is a few minutes old, the temperature is still hundreds of millions of degrees, but in order to form a stable, neutral atom, the temperature must drop below a few thousand degrees.
Sure, the Universe is expanding, which means its cooling as the wavelength of light within it stretches. But to stretch by that much — by a factor of about 100,000 — is going to take a lot of time, especially relative to the steps that came before.
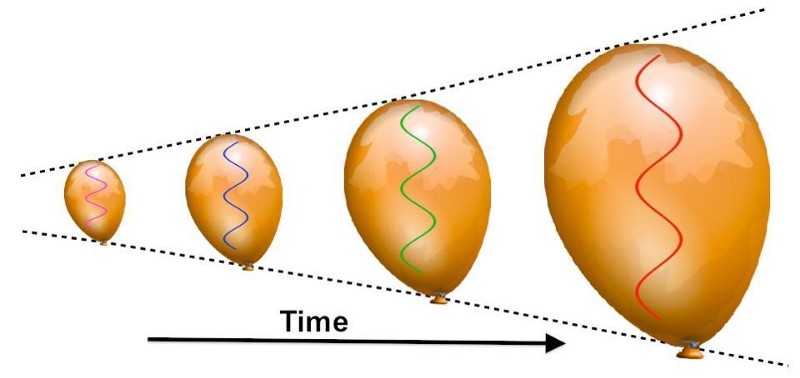
So the Universe waits. And as time goes on, it does expand and cool. As the minutes turn to hours and then to days, beryllium-7 starts to radioactively decay. By capturing electrons, it slowly transforms its way into lithium-7, and after the days turn into years, it’s virtually completely gone. As the years turn into decades, tritium radioactively decays (by electron and antineutrino emission) into helium-3. This transformation completes after roughly a century or two. And yet, still it’s far too hot to form a stable atom. So the Universe expands, cools, and gets less dense, all while the Universe continues to age.
As the centuries turn into millennia, the redshifting of these photons — which outnumber the other particles by about a billion-to-one — becomes so severe that they’ve lost almost all of their energy, having cooled down to just around 10,000 K: a far cry from what it once was. After a few tens of thousands of years, the radiation density then drops below the matter density, meaning that the Universe is now dominated by slow-moving matter, rather than radiation which moves at the speed of light.
With this critical change, gravitation now begins to pull the dark matter into clumps, which grow and grow, attracting more matter to them. Without enough energetic radiation to wash these clumps out, the Universe begins to form structure. The seeds of our cosmic web are starting to sprout.
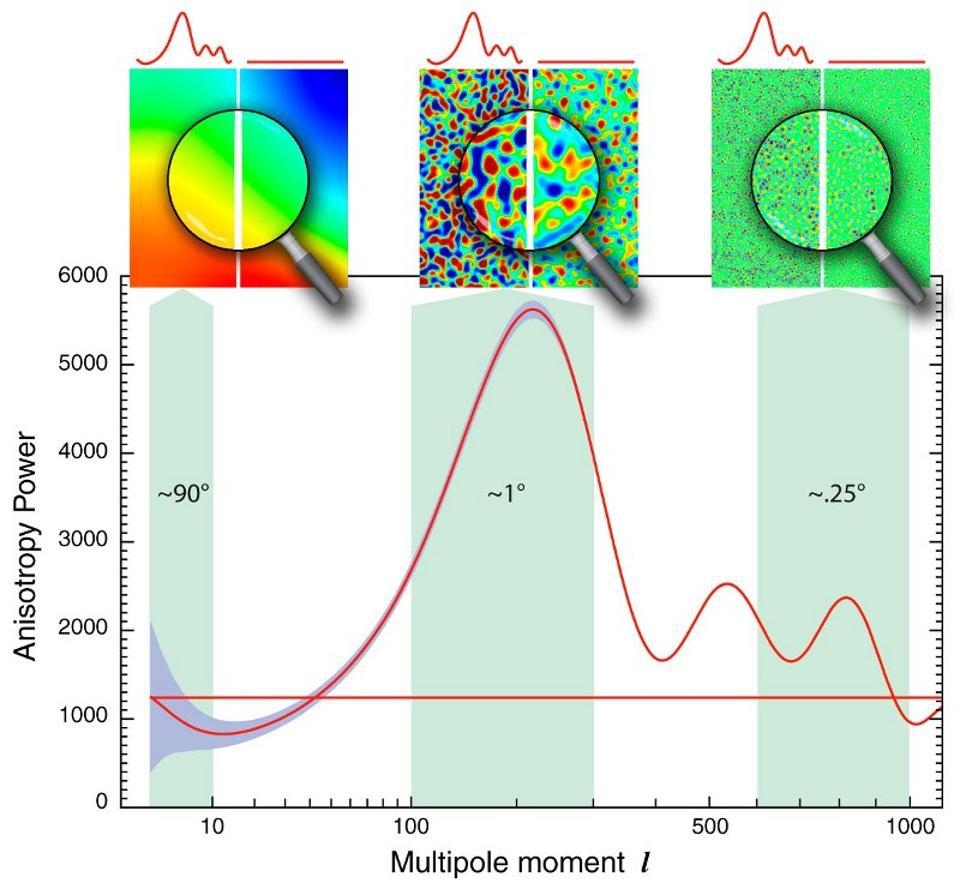
But even with all of those changes to the Universe, conditions are still too hot to form neutral atoms. Every time an electron successfully binds with an atomic nucleus, it does two things:
- It emits an ultraviolet photon, because atomic transitions always cascade down in energy levels in a predictable fashion.
- It gets bombarded by other particles, including the billion-or-so photons that exist for every electron in the Universe.
And during these stages, which many cosmologists call the “late early Universe,” at an age of tens of thousands of years old, there are:
- enough photons,
- that each have enough energy,
- for each and every “attempted atom” that we’d want to form,
so that pretty much as soon as an electron binds to a nucleus — whether a free proton or a heavier nucleus — it immediately gets blasted back apart. Even though most of the photons that exist aren’t of sufficient energy to blast those atoms apart, the fact that there are over a billion photons for each atom means that even a one-in-a-billion photon with enough energy can do the job of keeping the Universe ionized.
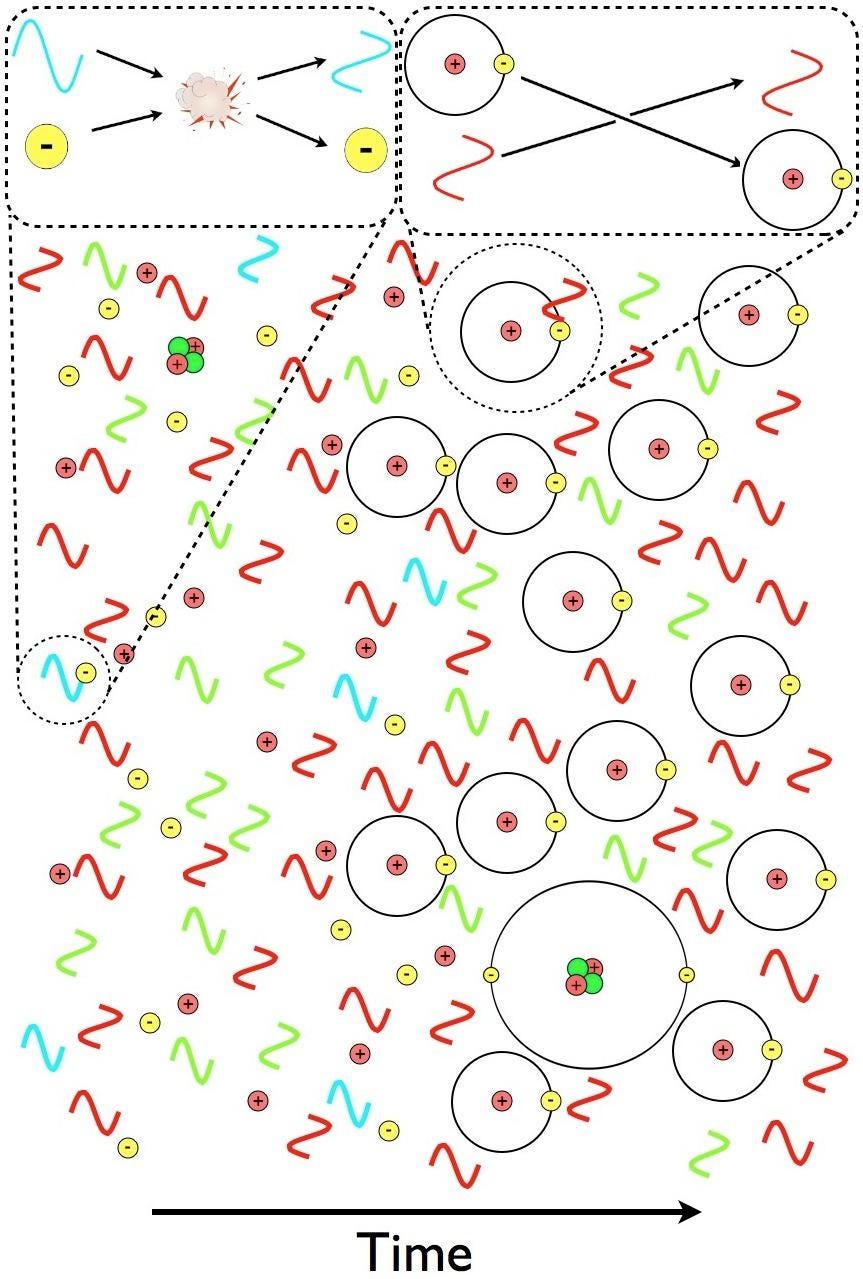
But something starts to change when the Universe reaches around 300,000 years of age. The background photons that are part of the leftover Big Bang have finally become too cool to immediately kick electrons off of their nuclei. There are still a few of those very high energies, but there are now fewer such photons than there are electrons in the Universe. The number of photons that are present with enough energy to ionize a neutral atom are fewer than the number of neutral atoms that are attempting to form. After enough time the energy balance of the Universe has finally “tilted” in the opposite direction.
This means that neutral atoms can begin to form, at last! But now there’s another problem for them to reckon with: can they remain as stable, neutral atoms, or will something come along to ionize them again?
This is not a trivial matter. Whenever you form a stable, neutral atom, the electrons cascade down the various energy levels. As they transition down to the ground state, each atom winds up emitting one ultraviolet photon. Those photons then continue on, in a straight line, until they encounter another neutral atom, which they then ionize. Even though a small number of neutral atoms are made at this point, they don’t stay neutral for long.
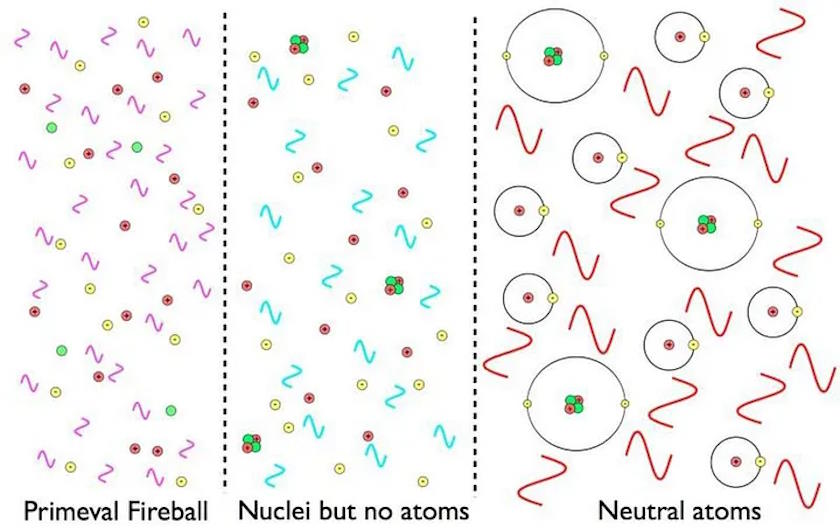
You might simply think that all we need to do is wait for longer amounts of time. After all, if you wait for long enough, then eventually these ultraviolet photons will travel through the expanding Universe for great enough amounts of time that they’ll redshift, and no longer interact (because they’re not at the right wavelength) with the electrons within each neutral atom. Once those ultraviolet photons can’t be absorbed by a ground-state atom, those atoms won’t get excited up to almost-ionized energies, causing them to remain neutral. It’s a very intuitive way to think about how neutral atoms could still yet cumulatively form within the expanding Universe.
And while it’s true that this is an effect that does indeed occur, it’s not the dominant pathway that leads to the majority of neutral atoms within our Universe. In fact, it’s only responsible for a few percent of the neutral atoms that come to form after the hot Big Bang. There’s another effect that comes in, instead, that dominates. It’s based on an extremely rare quantum transition, but given all the atoms in the Universe and the more-than-100,000 years it takes for atoms to finally and stably become neutral, it’s an incredible and intricate part of the story.
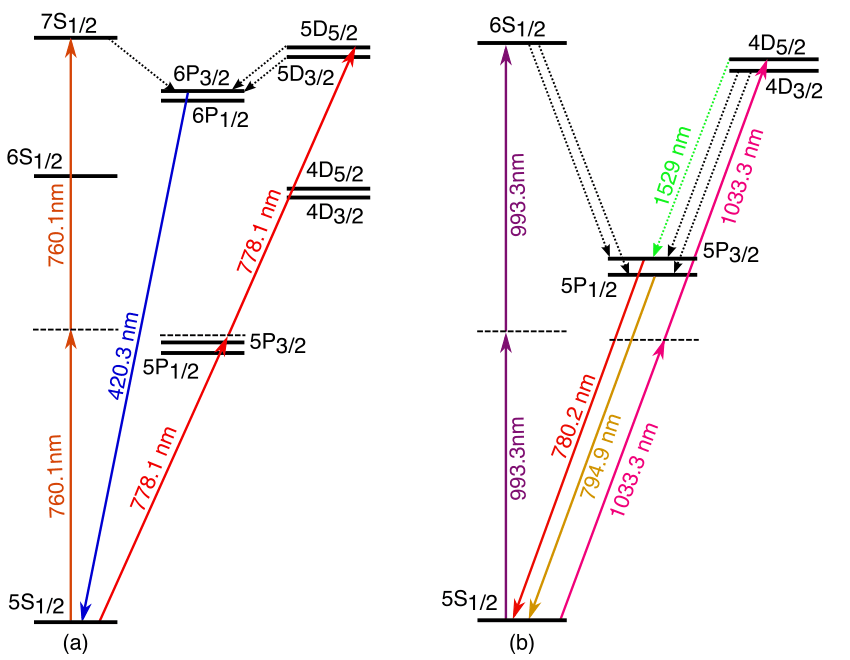
Most times, in a hydrogen atom, when you have an electron occupying the first excited state, it simply drops down to the lowest-energy state, emitting an ultraviolet photon of a specific energy: a Lyman alpha photon. That’s the most common occurrence, and it happens about 99,999,999 times out of every 100 million transitions.
But the “other” 1 time in every 100 million transitions, the drop-down will occur through a different pathway, instead emitting two lower-energy photons at once. This is known as a two-photon decay or transition, and it’s this process that’s primarily responsible for the Universe becoming neutral.
Here’s why that’s important. Whenever you emit a single photon, it almost always collides with another hydrogen atom, exciting it and putting it in an energized state: precariously close to its reionization energy. 99.999999% of the time, reionization of a new atom is going to occur because you made another atom of neutral hydrogen elsewhere. But when you emit two photons, it’s extraordinarily unlikely that both photons will hit an atom at the same time, meaning that it’s extraordinarily likely that you’ll net one additional neutral atom from this process.
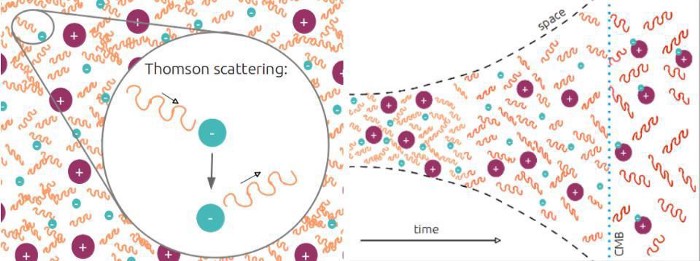
The rest is history. Sure, it takes more than 100,000 years for the process of making neutral atoms to complete — from the time we form our first net neutral atom until the final free electron and proton combine to make the final neutral hydrogen atom — but this is the known process by which the Universe does it. The all-important two-photon transition, rare though it is, is the main process by which neutral atoms first form. It takes us from a hot, plasma-filled Universe to an almost-equally-hot Universe filled with 100% neutral atoms.
Although we say that the Universe formed these atoms 380,000 years after the Big Bang, that’s only the “average” time at which the atoms in the Universe become neutral. In truth, the formation of neutral atoms was actually a slow, gradual process that took about 100,000 years on either side of that figure to complete. Once the atoms are neutral, there is nothing left for the Big Bang’s light to scatter off of: they scatter most efficiently off of low-mass, high-charge particles (i.e., electrons). Once atoms become neutral, the leftover radiation from the Big Bang simply streams freely: which is the origin of the CMB, or Cosmic Microwave Background.
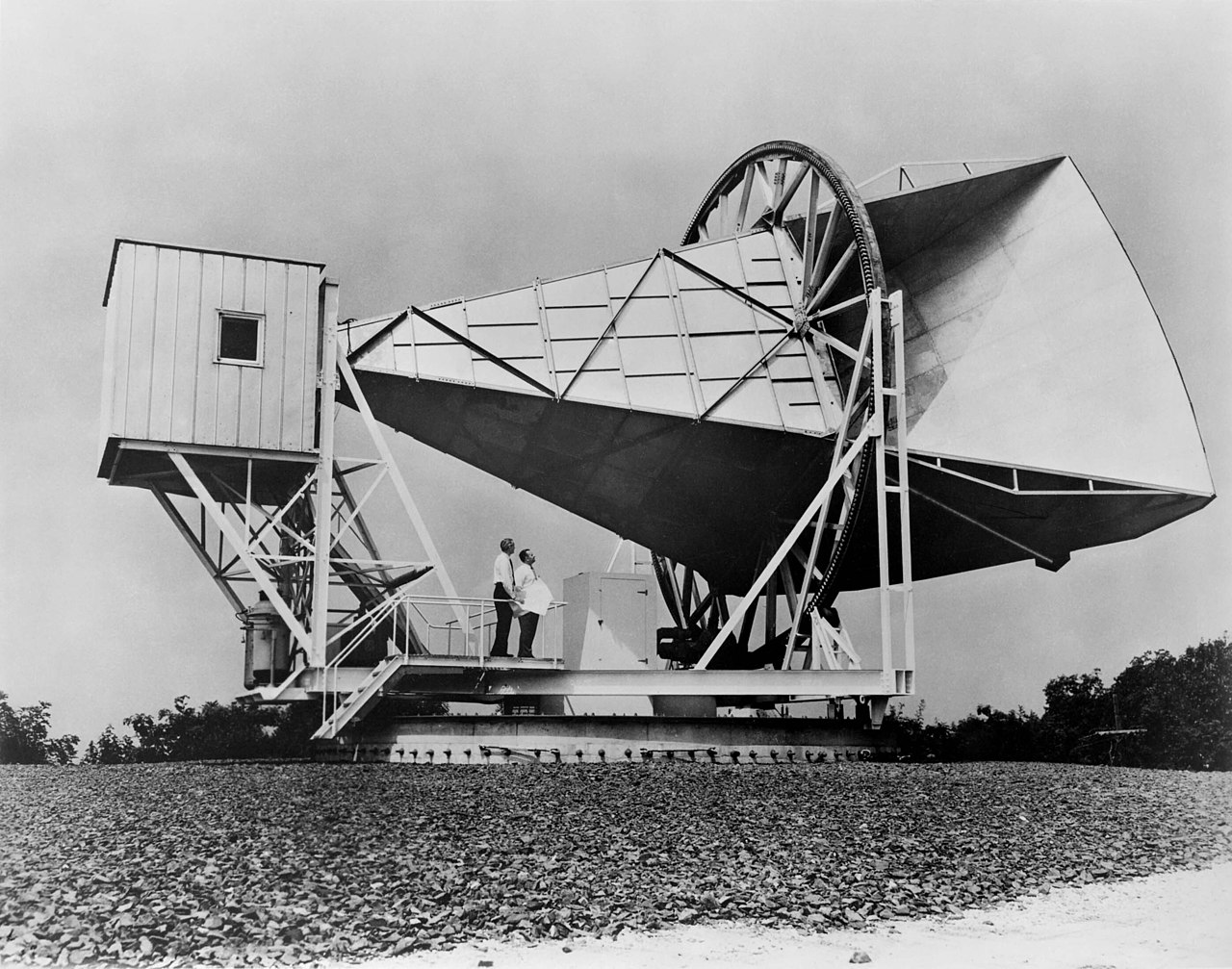
We first detected this light beginning in 1964, confirming the Big Bang and ushering in the era of modern cosmology. From our best observations at present, we’ve been able to confirm this spectacular picture, even measuring the depth and thickness of the last-scattering surface from this time. We’ve also measured the energy spectrum of the photons left over from the Big Bang, confirming them not only to be a blackbody (as predicted), but the most perfect blackbody ever measured, anywhere in the Universe. On large, cosmic scales, the evidence for how neutral atoms were made cannot be ignored.
And similarly, there’s more evidence for this to be found right here on Earth. Two-photon transitions have been verified here in terrestrial laboratories, and what we’ve observed represents a spectacular agreement between our theoretical predictions and what actually occurred in the Universe’s distant past. It took around half-a-million years for the Universe to finally, completely form neutral atoms, all while gravitation began to pull the Universe together into clumps. The cosmic story that would lead to us, at last, was ready to continue on to the next phase: where neutral atoms can finally lead to all that will come to exist down the line as part of the cosmic story.